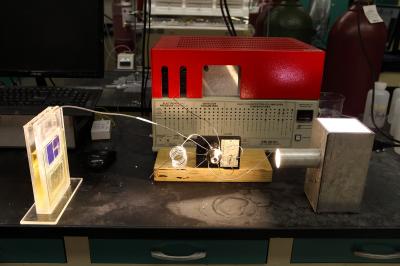
New solar cell captures CO2 and sunlight to create real fuel
There’s a new kind of solar cell on the block — one that’s taking on extra work. Instead of capturing sunlight and converting it into electricity, this new cell converts atmospheric carbon dioxide directly into usable hydrocarbon fuel, using only sunlight for energy, in an inexpensive and efficient way.
The game-changing cell was engineered by researchers at the University of Illinois at Chicago and a provisional patent application has been filed for the technology.
How it works
While conventional solar cells convert sunlight into electricity that needs to be stored in heavy batteries, the new device does the work of plants, converting atmospheric carbon dioxide into fuel. Therefore, a solar farm these “artificial leaves,” as they can be referred to, could remove significant amounts of carbon from the atmosphere and produce energy-dense fuel efficiently.
“The new solar cell is not photovoltaic — it’s photosynthetic,” says Amin Salehi-Khojin, assistant professor of mechanical and industrial engineering at UIC and senior author on the study. “Instead of producing energy in an unsustainable one-way route from fossil fuels to greenhouse gas, we can now reverse the process and recycle atmospheric carbon into fuel using sunlight,” he said.
The same way that plants produce fuel in the form of sugar, the artificial leaf delivers synthesis gas (syngas), a mixture of hydrogen gas and carbon monoxide. Syngas can be burned directly, or converted into diesel or other hydrocarbon fuels.
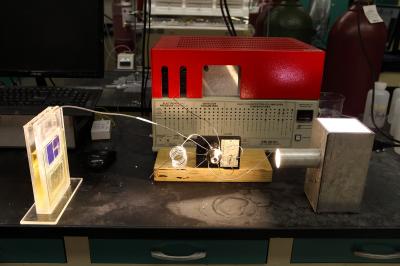
This technology, with its novel ability to turn CO2 into fuel at a cost almost that of a gallon of gasoline, could potentially do away with fossil fuels completely.
Researchers have been working for some time to develop different catalysts that would create these reduction reactions, chemical reactions that convert CO2 into burnable forms of carbon, but so far such reactions have been inefficient and rely on expensive precious metals such as silver, according to researcher Salehi-Khojin.
“What we needed was a new family of chemicals with extraordinary properties,” said Salehi-Khojin.
Salehi-Khojin and the team focused on a family of nano-structured compounds called transition metal dichalcogenides, or TMDCs, as catalysts, and paired them with an unconventional ionic liquid as the electrolyte inside a two-compartment, three-electrode electrochemical cell.
The best-performing ended up being nanoflake tungsten diselenide.
“The new catalyst is more active; more able to break carbon dioxide’s chemical bonds,” said Mohammad Asadi, UIC postdoctoral researcher and first author on the Science paper.
According to Asadi, the new catalyst is 1,000 times faster than noble-metal catalysts and about 20 times cheaper.
Other researchers have used TMDC catalysts to produce hydrogen by other means, but not by reduction of CO2, since they could not withstand the reaction itself.
“The active sites of the catalyst get poisoned and oxidized,” said Salehi-Khojin. T
The researchers combine ethyl-methyl-imidazolium tetrafluoroborate, mixed 50-50 with water, which results in a co-catalyst that preserves the catalyst’s active sites under the harsh reduction reaction conditions.
The artificial leaf comprises two silicon triple-junction photovoltaic cells that measure 18 square centimeters to harvest light; the tungsten diselenide and ionic liquid co-catalyst system on the cathode side; and cobalt oxide in potassium phosphate electrolyte on the anode side.
When 100 watts per square meter of light (about the average intensity reaching the Earth’s surface) energizes the cell, hydrogen and carbon monoxide gas bubble up from the cathode, while free oxygen and hydrogen ions are produced at the anode.
“The hydrogen ions diffuse through a membrane to the cathode side, to participate in the carbon dioxide reduction reaction,” said Asadi.
The technology will be adaptable on a large-scale, like for solar farms, but also for smaller-scale applications. The researcher say it may even be useful on Mars in the future, since the planet’s atmosphere is mostly carbon dioxide.
“This work has benefitted from the significant history of NSF support for basic research that feeds directly into valuable technologies and engineering achievements,” said Robert McCabe, NSF program director.
“The results nicely meld experimental and computational studies to obtain new insight into the unique electronic properties of transition metal dichalcogenides,” said McCabe. “The research team has combined this mechanistic insight with some clever electrochemical engineering to make significant progress in one of the grand-challenge areas of catalysis as related to energy conversion and the environment.”
Comments are closed, but trackbacks and pingbacks are open.