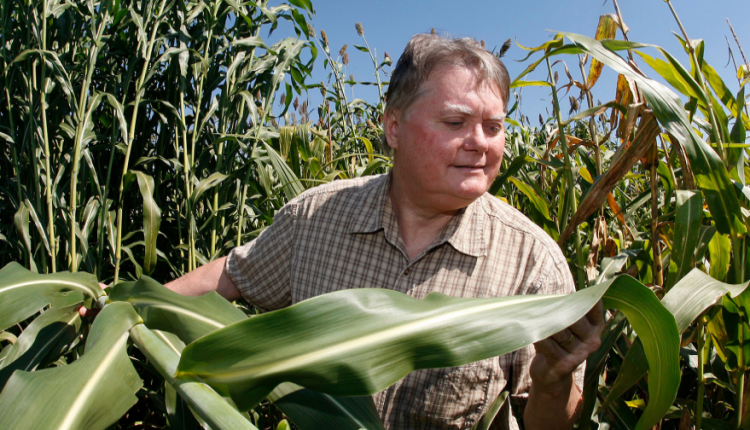
The need to lessen our reliance on fossil fuels by using biofuels has been fraught with controversy. Dealing with fossil fuel lobbyists has not been the only challenge. The food vs. fuel debate over using arable land to grow biomass capable of being converted to biofuels has also been a barrier to adoption. Now, the focus is shifting to increase production of second-, third- and fourth-generation biofuels.
But second-generation biofuels made from non-food biomass such as switchgrass, biomass sorghum, and corn stover ran into problems due to difficulty in efficiently converting biomass to fuel.
Lignin, a complex compound in cell walls, blocks access to plant carbohydrates that could be cleaved into sugars and then fermented into biofuels. The compounds that hold plant cells together, as well as their tightly packed cell clusters, also block access to sugars for fermentation into fuels.
Now, a team led by Purdue University has built on success in removing the lignin barrier to solve other cellular obstacles. Their findings, reported in the journals Plant Biotechnology Journal and Biotechnology for Biofuels, offer opportunities to significantly increase renewable biofuel production from crop waste products and bio feedstocks that could be grown on marginal lands.
“Lignin is no longer a problem. We have a way of removing it and making useful products from it, as well as getting access to plant carbohydrates for production of biofuels,” said Nick Carpita, a Purdue professor in the Department of Botany and Plant Pathology.
Purdue’s C3Bio Energy Frontier Research Center has worked for more than a decade to tailor bioenergy crop species for chemical conversion to liquid hydrocarbon fuels like gasoline or jet fuel. Led by Maureen McCann, the C3Bio team has explored the obstacles besides lignin that must be overcome to make the carbohydrates more accessible for fuel production.
“Removing lignin didn’t eliminate all the issues of biomass recalcitrance,” McCann said. “We needed to look at factors that made woody biomass difficult to degrade beyond lignin, and in its absence.”
Former Purdue chemist Mahdi Abu-Omar had discovered that using a nickel-carbon catalyst was an inexpensive and effective method for removing lignin without degrading the plant’s carbohydrates. Even with lignin removed, however, the Purdue team had to find ways to break the tightly connected plant cells apart so that chemical catalysts or yeasts used in the biofuel refining process could do their jobs.
Genetically modified poplars with altered lignin structure
Plant biologists Clint Chapple and Rick Meilan have now developed a genetically modified poplar tree with altered lignin structure. Lignin is made of three basic building blocks called monolignols—guaiacyl (G), p-hydroxyl phenol (H), and syringyl (S). One of the trees developed by Chapple and Meilan contains greater than 90% S-lignin, which has weaker bonds with plant carbohydrates.
Other poplar trees were also genetically modified to allow for easy breakdown of rhamnogalacturonan, a pectin-like substance in the middle lamella, the zone that glues the walls of plant cells together. Meilan and McCann overexpressed genes that control production of rhamnogalacturonan lyase (RG-lyase), an enzyme that breaks down rhamnogalacturonan, removing the connections between cells.
“Although rhamnogalacturonan is present at only 2% of the mass of the cell wall, removing it allows you to deconstruct the biomass particles into smaller clusters of cells, and that can have real energy savings when trees are being shredded to particles for any conversion process,” McCann said. “Lignin is also deposited in the middle lamella, but removing only the lignin using the nickel-carbon catalysis didn’t allow the cells to become unglued.”
With all the lignin removed from Chapple and Meilan’s poplar through the nickel-carbon catalysis, the team treated poplar wood particles with trifluoroacetic acid to loosen the tightly packed crystalline cellulose and its aggregation into large bundles in plant cell walls.
The trifluoroacetic acid causes the cellulose to swell, making it easier to access the glucose molecules present in the cell walls for fermentation to ethanol. Or, using other chemical catalysts discovered by the C3Bio team, the cellulose and other carbohydrates can be converted to platform chemicals, such as hydroxymethylfurfural and levulinic acid, which are substrates or precursors for liquid hydrocarbon fuels.
For now, the engineered poplars cannot be grown commercially as a bio feedstock because they’re genetically modified organisms. They would need costly and difficult-to-obtain federal government approvals to grow these trees for any purpose other than research. But the knowledge gained from them could be used in other crops modified through gene-editing CRISPR technology.
“We now know how to disassemble the cell walls to produce various products, including transportation fuel,” Meilan said. “What we’re doing with poplar can help inform what’s being done with other cellulosic feedstocks derived from corn stalk residues, or biomass sorghum and switchgrass.”
CRISPR could also be used to modify plants like sorghum and generate not only biofuels, but also chemicals from lignin and other compounds removed from plant cell walls.
The research was supported by the Center for Direct Catalytic Conversion of Biomass to Biofuels (C3Bio), an Energy Frontier Research Center funded by the U.S. Department of Energy. The Purdue researchers involved with the work are all members of the Purdue Center for Plant Biology.
Source: Purdue University