The Battery Innovations That Could Help Spread Renewable Energy
In the 1940 novella Revolt On The Tenth World, science fiction author, Edmond Hamilton described ‘solid power … the most super-valuable substance in the Solar System.’
Solid power is “compressed energy ‘frozen’ by temporary transformation into artificial atoms. Trillions of units of power … compressed thus into an inch-square cube.”
Hamilton and other sci-fi writers throughout the life of the genre have fantasized about similar compact energy sources.
They’ve had to. What else could make their starships fly, their laser guns blast and their communicators buzz?
The closest we come to these powerhouses in reality are rechargeable batteries. Our ‘communicators’ – cell phones, tablets, laptop computers and similar devices – rely on the ‘solid power’ in these batteries to keep us connected.
Similarly, hybrid and electric vehicles use much larger versions to transport their occupants to far-off destinations – or at least across town.
Currently, lithium-ion batteries represent the most energy-dense batteries available for practical use, storing hours of electricity for most devices in a relatively compact, lightweight size. But Sri Narayan, professor of chemistry at USC Dornsife and co-director of the USC Loker Hydrocarbon Research Institute, wants to do better.
Power up
First inspired to pursue chemistry by a high school teacher, Narayan went on to attend graduate school in India, where he began studying power cells based on magnesium, an element that is readily available from sea water.
After earning his Ph.D., he wanted to continue his work with batteries, but by then lithium-based technology was emerging.
NASA’s Jet Propulsion Laboratory was hot on this trend, since lithium could provide a great deal of power while adding little weight to a spacecraft (a dream come true for sci-fi enthusiasts and scientists alike).
Narayan joined JPL in 1992 and spent nearly 20 years advancing various types of batteries and fuel cells, including the lithium-ion battery technology used on the Mars Spirit, Opportunity and Curiosity rovers.
He came to USC Dornsife in 2010 to expand his work on batteries into new areas. “USC has been very good because I have good students, I have good support from the faculty and it’s a very collaborative environment,” he said.
Among other pursuits, Narayan is working on a way to bring lithium-sulfur batteries into widespread use. These batteries pack two to three times more energy than their lithium-ion counterparts.
They’re also less prone to overheating, which means they don’t need the complex control systems that keep lithium-ion batteries from exhibiting one of their more inconvenient tendencies – erupting in flame when overcharged or overtaxed.
Increased safety, and the fact that sulfur is abundant and cheap, means lithium-sulfur power packs would be much less expensive to produce than lithium-ion batteries.
Unfortunately, lithium-sulfur batteries don’t hold a charge or withstand recharging worth a hoot. “Lithium-sulfur, today, is at a hundred cycles. That’s all. It’s pathetic,” Narayan said.
Given that most people charge their phones daily, this means replacing the battery within three months. Lithium-ion batteries generally last two to three years, and sometimes more. The short life of the lithium-sulfur batteries stems from the tendency for sulfur ions, called sulfides, to cling to one another and then escape from the positive electrode (the cathode).
The polysulfide ions flee to the negative electrode (the anode), where they grab electrons and head back to the cathode.
In this ‘polysulfide shuttle,’ many of the sulfide ions react with lithium ions to form lithium-sulfide, which is insoluble and utterly useless to the battery.
Both discharging and recharging the battery accelerate this shuttling process, degrading the cathode and anode until the battery goes kaput.
Narayan and Ph.D. student Derek Moy, however, developed a way to overcome the polysulfide shuttle and give these batteries longer lives.
MCM
Their solution is called a ‘mixed conduction membrane,’ or MCM.
This thin, nonporous material conducts lithium ions but blocks the polysulfide reactions that degrade the battery. Batteries with the MCM lasted through as many as 500 cycles with no loss of charge capacity, Narayan said.
He thinks that may be enough for cell phones, at least.
“Every couple of years you’re probably going to get a new phone anyway,” he said. “Five hundred cycles is plenty.”
While Narayan cautions that the technology is in its infancy and needs more research before they gain popular use, he thinks the advantages of lithium-sulfur – inexpensive, abundant materials and more energy per unit weight – could soon benefit handheld electronics and eventually vehicles.
“Lithium-sulfur will probably find its place in cell phones before it makes it into cars. That’s a natural projection,” he said.
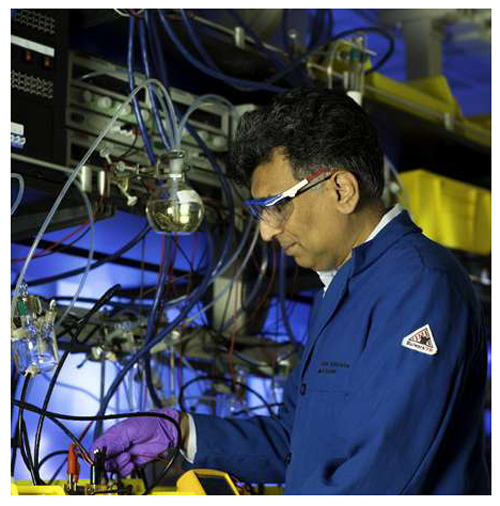
But there’s another level of need when it comes to batteries – something on a much larger scale.
Gridlock
Just off Interstate 15, about five miles west of the California-Nevada border in the far northeast reaches of San Bernardino County, thousands of mirrors glitter in the dry desert heat, focusing the day’s unrelenting sunshine onto three colossal towers in their midst.
The focused light and heat converts thousands of gallons of water within the monolithic structures to steam. That steam drives a turbine that produces – in theory, at least – an average of about 2.5 billion watt-hours of renewable electricity for power-hungry customers each day.
At night it produces nothing. Solar energy farms throughout the country – and across the globe – face this issue. When the sun disappears at night or slips behind dark clouds, the fields of solar collectors have little or no light energy to gather and convert to heat or electricity.
The same is true for wind farms, which rely on the motion of air moving through enormous windmill blades to keep the electricity flowing into the electrical grid.
When the winds calm, the blades stop, and the electrons stop moving. Batteries may provide a solution, storing excess renewable energy and feeding it to the grid when needed.
The idea has been around for years, but it remains problematic for a number of reasons. For Brent Melot, assistant professor of chemistry, it boils down to materials science—finding the resources that can fulfill the need safely and at reasonable cost.
Like Narayan, Melot focuses much of his research on advancing battery technology, developing efficiency and life for lithium-based units.
Current lithium-ion batteries, he said, could store enough power in a reasonable amount of space, but there are safety concerns. Batteries large enough to store enough energy to supply the grid would require sophisticated (read ‘expensive’) systems to ensure they don’t overheat or malfunction.
Also, they contain cobalt and nickel, which are exorbitantly costly. Oh, and both are toxic. “Cobalt ore right now costs about $46,000 per metric ton,” Melot said.
Nickel rings up at about $10,000 per metric ton. Lithium itself is about the same. In addition, these metals are available from limited locations on the planet, making their availability vulnerable to changes in the geopolitical climate.
Melot suggests, more abundant and readily accessible materials would be ideal – something like iron. “If we can find chemistries that are actually based on iron, iron ore is $90 per metric ton,” he said. It’s also nearly ubiquitous, virtually eliminating political concerns. And it won’t foul up the local landfill.
It so happens that Narayan is working on a couple of battery solutions addressing safety and cost, one of which produces electricity through the oxidation of iron in the presence of air.
Aside from reducing costs significantly, these iron-air batteries would be much safer, containing no corrosive or flammable solvents or dangerous heavy metals.
Iron-air batteries have been around for decades, but they suffered from extreme inefficiency, losing half their power to a side reaction that produces hydrogen.
Narayan and his colleagues managed to reduce the loss to just four percent, bringing the technology much closer to practical use.
That technology is now in the hands of a company that aims to bring it to market.
Go with the flow
Narayan has also been working on a radical idea called a redox flow battery. Like their iron-air cousins, flow batteries have been under investigation for decades.
Narayan, however, has been taking a new angle, working to find and use materials that will improve both the efficiency and stability of these systems while using safer materials. In redox flow batteries, electrons move from a tank of electrochemical solution, through the load (a washing machine or light bulb) to another tank of solution.
One advantage is that the batteries don’t age the way solid batteries do; there are no electrodes to break down over time. Perhaps more important, though, is the system’s flexibility: The solutions can undergo recharging – by a renewable energy source, for instance – or they can simply be replaced with fresh, fully charged solutions.
Concentrating on sustainability, Narayan has focused on developing flow batteries that use water-soluble organic compounds commonly found in plants.
“These compounds are essentially nontoxic and won’t catch fire the way solvents in lithium batteries can,” he said. They’re also simple and inexpensive, and they’re efficient, lasting a very long time. Professor and Chair of Chemistry G. K. Surya Prakash collaborates with Narayan in identifying and synthesizing the water-soluble, electroactive organic compounds for the flow batteries.
Director of the Loker Hydrocarbon Research Institute and holder of the George A. and Judith A. Olah Nobel Laureate Chair in Hydrocarbon Chemistry, Prakash touts the Earth-friendly nature of Narayan’s flow batteries.
“Water-based organic flow batteries are game changers,” Prakash said. “They use very little or no metal, the organic materials used are easily derived from earth-abundant, carbon-based resources, and they can also be made from recycled carbon dioxide, making them renewable.”
While redox flow batteries haven’t yet progressed the way iron-air batteries have, Melot puts his money on them as a solution for large-scale use.
“[Narayan’s] stuff, I think, is going to be the answer for grid level. It’s really innocuous, scales very easily and does the job,” he said.
Whether iron-air or redox flow technology, Narayan believes that sustainable energy storage with batteries will be the key to the ultimate success of renewable energy.
“It’s just not possible to do anything that isn’t sustainable,” he said, “and I view the field of electro-chemistry as being pivotal in … the future use of renewable energy. Make chemicals; make electrons from chemicals; store the electrons. All these are possible with electro-chemistry.”
Comments are closed, but trackbacks and pingbacks are open.