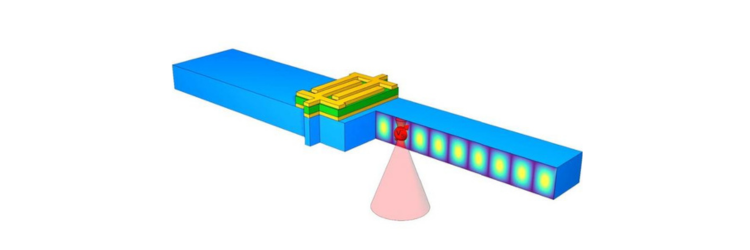
Using Sound for Device Test and Qubit Control
Acoustic resonators are ubiquitous. Smartphones, for example, have bulk acoustic resonators to filter out noise that could degrade an RF signal. Wi-Fi and GPS systems use the filters as well.
Unfortunately, these resonators degrade over time, and monitoring and analyzing the degradation was not possible—until now. Researchers at the Harvard John A. Paulson School of Engineering and Applied Sciences (SEAS), with OxideMEMS Lab at Purdue University, have developed a system using atomic vacancies in silicon carbide to measure the stability and quality of acoustic resonators. The vacancies can also be used for acoustically controlled quantum information processing to manipulate embedded quantum states. The research was published in Nature Electronics.
Silicon carbide is a common material for MEMS, including bulk acoustic resonators. It has high performance; however, crystal growth and resonator manufacturing defects such as roughness, tether stress, and micro-scale craters cause stress-concentration regions inside a MEMS resonator. Powerful, expensive X-rays, such as the broad-spectral X-ray beam at the Argonne National Lab, are the only way to monitor the resonators. That isn’t helpful in a foundry where the devices are made.
Silicon carbide has naturally occurring defects in which an atom is removed from the crystal lattice, creating a spatially local electronic state whose spin can interact with sound waves through material strain generated by an acoustic resonator. When the waves move through the material, they put mechanical strain on the lattice, potentially flipping the spin of the defect. Changes in the spin state can be seen by shining a laser through the material.
The defects are the size of single atoms, the information is very local, and it’s possible to map out the acoustic waves inside the devices. The map can point to where and how the system may be degrading or not operating optimally.
Defects in silicon carbide can also be qubits within a quantum system. The team demonstrated that they could control spin by mechanically deforming the material with acoustic waves for control, similar to approaches using alternating magnetic fields.